Pions form a new kind of helium
11 May 2020
The ability to make artificial atoms containing exotic particles in place of electrons is giving physicists a new way of probing fundamental interactions. Now, researchers have created and interrogated a novel kind of helium atom in which one of the electrons is replaced by a sub-atomic particle known as a pion. The work could shed light on the nature of both pions and neutrinos – tiny, neutral particles for which certain attributes, including mass, remain relatively poorly understood.
Among the particles previously used to make these unusual atoms is the muon, which is about 200 times as massive as the electron but otherwise has identical properties. In 2010 Randolf Pohl and colleagues at the Max Planck Institute of Quantum Optics (MPQ) in Garching, Germany, carried out spectroscopic measurements on muonic hydrogen. They used their data to great effect, calculating a value for the proton’s charge radius that was completely at odds with the then-accepted value and forcing other groups to try to resolve the discrepancy.
Creating atoms containing pions is more difficult. Pions are mesons, meaning that they consist of a bound quark and anti-quark. When fired at high speed into a dense target, some of them will form atoms. But they also create lots of background particles, which makes it hard to pick out the atoms. What’s more, pionic atoms, like the pions themselves, have very short lifetimes, which has made it difficult excite them with a laser beam and measure their internal transition frequencies.
Long-lived atoms
Masaki Hori, also at the MPQ, and colleagues have now overcome this barrier by creating relatively long-lived atoms of pionic helium. In an experiment at the Paul Scherrer Institute in Switzerland, they used an intense beam of protons to generate negative pions in a carbon target, then collided the pions with atoms of superfluid helium-4.
Most of the incoming pions slam directly into a helium nucleus, causing the helium to split into a proton, neutron and deuteron within around a picosecond (10–12 s). But roughly 2% manage to displace one of helium’s two electrons and enter a weakly-bound orbit around the nucleus. The bound pions maintain this orbit for several nanoseconds (10–9 s), as they are protected from the effects of thermal collisions with other atoms by both the remaining electron and the very cold target, which is held at just a couple of degrees above absolute zero.
Left to their own devices, the pionic helium atoms will eventually split and generate fission products that can be picked up by detectors positioned around the target. The problem is that the signal from these fission products gets swamped by the signal from the nucleons created by the remaining 98% of incoming pions. The pions arrive in pulses every 20 ns, thanks to the 50 MHz frequency of the accelerator cavities, and they produce big peaks in the detector counts. In contrast, the spontaneous decays of the metastable pionic atoms produce an unobservable smooth background.
Transition frequency measurement
This is where the laser spectroscopy comes in. As they report in Nature, Hori and colleagues synchronized an infrared laser with the pion beam so that it fires a 0.8ns-long light pulse about halfway between each successive pulse of pions. This causes some of the pions in their respective nuclei to drop to a lower orbit and eject the remaining electron, which they do in a few picoseconds, before crashing into the nucleus. Although this process yields just three pionic atoms per hour above the background, that is enough to register a significant peak in the detector count.
By scanning the frequency of their laser beam, the researchers established that the transition they were studying occurred at 183,760 GHz. They point out that the accuracy of this measurement is limited by the many collisions with helium atoms, each of which shifts the frequency slightly, and the broad but easy-to-identify atomic resonance that they used. They hope eventually to use their experiment to set a new, more accurate value for the mass of the negative pion (currently known to just six decimal places), and propose both thinning out the target and studying atomic transitions with narrower linewidths.READ MORE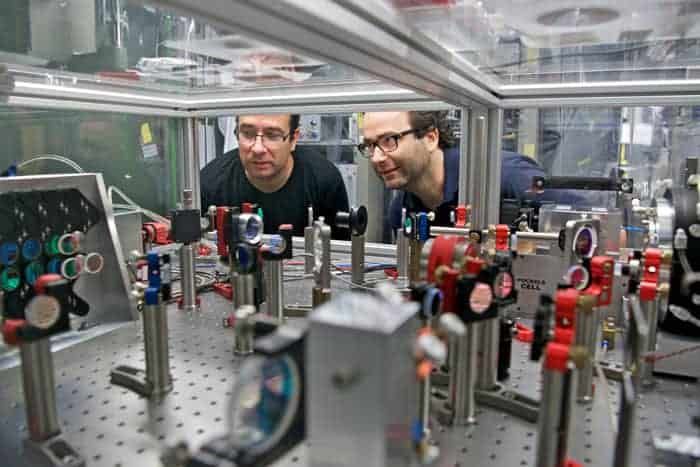
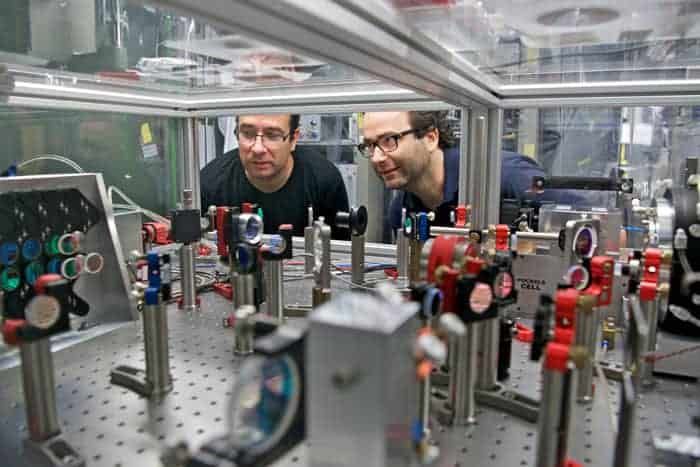
Improving mass accuracy
In a commentary written to accompany the research, Niels Madsen of Swansea University in the UK points out that Hori’s group has previously achieved record precision for the antiproton-to-electron mass ratio by replacing one electron in a helium atom with an antiproton. However, he says that improving the negative-pion mass accuracy will be tougher, partly because a lower helium density means less signal. Nevertheless, he argues that, with persistence, the accuracy could improve by a factor of 10–100. “The experiment thus paves the way to fresh insights into the fundamental constituents of nature,” he writes.
Hori points out that shoring up the pion’s mass could also lower particle physicists’ current upper limit on the mass of the muon antineutrino, given that pions decay into muons (the mass of which is very well known) and neutrinos. “The muon neutrino mass can be estimated by indirect methods much more precisely,” he says. “But it is always nice to have a direct laboratory determination.”
from physicsworld.com 9/10/2020
Δεν υπάρχουν σχόλια:
Δημοσίευση σχολίου