Cryogenics makes the European Spallation Source a hot property in neutron science
06 Jul 2020
Cryogenics is a core enabling technology at the European Spallation Source, a next-generation neutron-science facility under construction in Sweden. Joe McEntee talks to John G Weisend II, group leader for specialized technical services at ESS, about the secrets of success at ultralow temperatures

Large-scale neutron facilities – one of the mainstays of Europe’s “big science” infrastructure – are routinely used by researchers to understand material properties on the atomic scale, spurring advances across a spectrum of scientific discovery – from clean energy and environmental technology to pharma and healthcare, from structural biology and nanotech to food science and cultural heritage. Industry users, meanwhile, use neutrons to probe deep into engineering components, gaining unique insights into the stresses and strains that affect turbine blades, gas pipelines, fuel cells and the like.
Big science, of course, keeps thinking bigger – and neutron science is no exception. For the neutron user community, in fact, a decade of revolution rather than evolution is hoving into view as construction progresses on the European Spallation Source (ESS), a €1.84bn accelerator-driven neutron source in Lund, Sweden. When it comes online for user experiments in 2023, the ESS will be the world’s most powerful neutron source – between 20 and 100 times brighter than the Institut Laue Langevin (ILL) in Grenoble, France, and up to five times more powerful than the Spallation Neutron Source (SNS) in Oak Ridge, Tennessee, US.
John G Weisend II: “Big science is all about collaboration, so the number-one priority, from the off, is to have tight communication with your industry vendors and in-kind project partners.” (Courtesy: ESS)
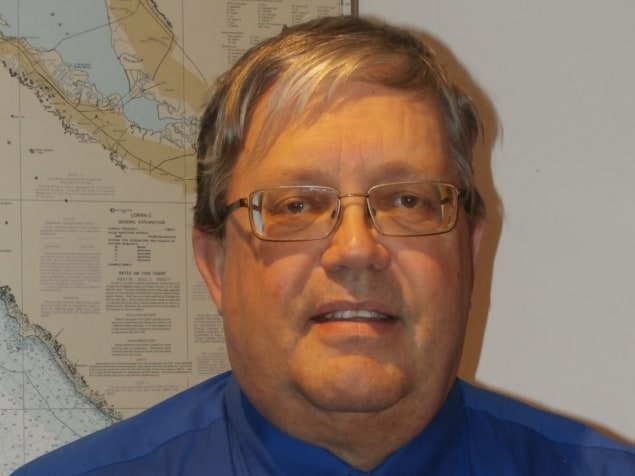
That big leap forward represents an industrial-scale undertaking, an amalgam of the most powerful linear proton accelerator ever built; a two-tonne, rotating tungsten target wheel (which produces neutrons via the spallation process); 22 state-of-the-art neutron instruments for user experiments; and a high-performance data management and software development centre (located in Copenhagen). John G Weisend II, group leader for specialized technical services at ESS, explains how liquid-helium cryogenic technologies – which enable production and maintenance of temperatures as low as 2 K – are equally fundamental to the ESS’s long-term scientific success.
What are the main building blocks of the ESS cryogenics programme?
There are three principal applications of helium cryogenics within the ESS. The proton linac’s superconducting RF cryomodules (responsible for the bulk of the particle acceleration) require cooling at 2 K, 4.5 K and 40 K, while the hydrogen moderator (surrounding the tungsten target that produces the neutrons) requires cooling via 16.5 K supercritical helium.
Elsewhere, many of the facility’s scientific instruments will rely on liquid helium to cool hardware like superconducting magnets or to provide low-temperature environments around the instruments and sample chambers themselves.
To meet these needs, we specified a system design with three distinct cryogenic refrigeration plants sharing a largely common recovery, purification and storage system (see “The headline take on ESS” below). After an open tendering process, Linde emerged as our chosen supplier for the accelerator cryoplant and target moderator cryoplant, with Air Liquide contracted to supply the test and instruments cryoplant as well as the recovery and purification system.
How did you approach that engagement with industry?
One of the challenges with a cryogenic system of this scale is the lead time – more than five years from the initial feasibility studies and the writing of the detailed technical specifications through to the on-site installation and commissioning at ESS. As such, it’s vital to get under way early with the cryoplant design studies and equipment procurement. Although the three ESS cryoplants are by no means off-the-shelf solutions, we were careful not to be overly innovative in terms of our requirements-gathering. Instead, the emphasis was on capital cost, best-in-class reliability and minimizing operating expenditure – chiefly through energy efficiency.
Keeping cool: two of the three ESS “cold-boxes” – the target moderator cryoplant (left) and accelerator cryoplant (right) – were supplied by Linde. (Courtesy: Ulrika Hammarlund/ESS)
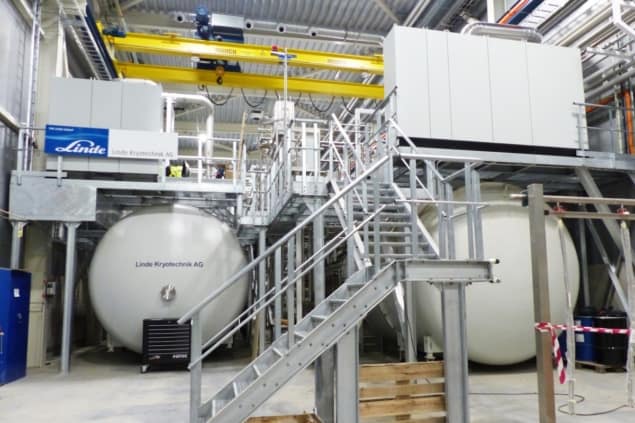
That commitment to sustainability is a unique aspect of the ESS project. What does this mean for the cryogenics programme?
One aspect of the ESS sustainability plan is to recover as much waste heat as possible – at least 50% – and supply it into the domestic hot-water heating system in the Lund metro area. Across all our cryogenics systems, we are recovering the heat deposited in the oil and helium coolers of the warm compressors as well as in the compressor motors.
Does the emphasis on sustainability extend to helium recovery and storage?
Helium is a finite resource and an industrial gas that’s subject to significant price fluctuations. With this in mind, all of our cryogenic operating cycles are closed, designed to limit the venting of helium into the atmosphere to a few, rare failure modes. Over the next five years, as we commission and put the ESS cryoplant through its paces, we expect to replace approximately 50% of our helium inventory each year. Beyond that, we aim to get down below 25% replacement levels per annum.
Many ESS project partners make “in-kind” contributions of equipment and personnel rather than direct cash investments from the member countries. How does this work in terms of the cryogenics programme?
A good example is the cryogenic distribution system, which connects the accelerator cryoplant to the cryomodules in the accelerator tunnel and, uniquely, is set up in such a way that we can independently cool down or warm up any of the cryomodules while keeping the others at their operating temperature. This capability allows us to repair a specific cryomodule in situ while keeping the others at cryogenic temperatures – the aim being to reduce downtime and improve the availability of ESS for the scientific users.
Unlike the rest of the cryogenic infrastructure, the distribution system is provided by in-kind partners, with the bulk of the work carried out by Wroclaw University of Science and Technology in Poland and its local industry partner Kriosystem; the remainder is handled by the Laboratoire de Physique des 2 Infinis Irène Joliot-Curie in France and its industry partner Cryo Diffusion. It’s worth noting that these relationships are very much in the spirit of scientific collaboration rather than contractor/subcontractor arrangements.
The long view: this section of the cryogenic distribution system in the ESS accelerator tunnel is an in-kind contribution from Poland. (Courtesy: Ulrika Hammarlund/ESS)
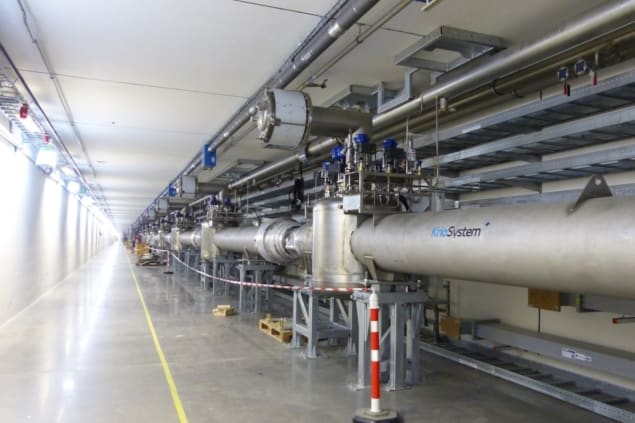
How has the ESS construction project been impacted by the coronavirus pandemic?
While most ESS staff are working from home, civil construction proceeds almost as normal and a significant amount of installation of technical infrastructure is still taking place on site. However, ESS depends on deliveries and interaction with our in-kind partners and suppliers, and we see that the supply chain has been affected by the pandemic.
In terms of the cryogenics deployment, current status is that the test and instruments cryoplant is fully commissioned and turned over to us, as is the target moderator cryoplant. The accelerator cryoplant has been commissioned to its highest-capacity cooling at 2 K. Working with Linde, we expect to complete the commissioning this summer. The last part of the cryogenic distribution system has been delayed, but we do expect to commission it in early 2021.
What lessons can other big-science projects learn from your experience at ESS?
We’ve done a lot of things right on the ESS cryogenics programme. Big science is all about collaboration, so the number-one priority, from the off, is to have tight communication with your industry vendors and in-kind project partners. Communication needs to work consistently well at all levels – from the director right down to the project engineers at the sharp end. We also carved out time on personnel and recruitment, building a world-class, 10-strong team of engineers, scientists, designers and technicians to manage and work with a diverse group of stakeholders.
On the flip side, one area to guard against is over-reach. A case in point is a project that we took on in-house and underestimated – building the warm-gas connections between the three cryoplants and cryogen storage systems. While we wound up getting the work done on time, it probably would have been prudent – and less stressful – to allocate more resource earlier. We share all of these lessons via regular conference presentations and exchange visits with our counterparts from other large-scale facilities.
What’s next for you and the ESS cryogenics team?
The pace is relentless and likely to be even more so once we get beyond the pandemic. Commissioning of the accelerator cryoplant and the cryogenic distribution system should be completed in 2020, while installation of the cryomodules in the linac tunnel should start at the end of the year and continue through 2021. The first cooldown of the ESS linac is planned for 2022.
The European Spallation Source: an overview
Fundamental principles
At the heart of the ESS is a linear accelerator that produces up to a 5 MW beam of 2 GeV protons, with the bulk of the acceleration generated by more than 100 superconducting RF cavities.
These protons strike a rotating tungsten target wheel to produce a beam of neutrons via a process known as nuclear spallation (i.e. the impact on the tungsten nuclei effectively “spalls” off free neutrons).
The resulting neutrons pass through a supercritical hydrogen moderator (at about 17 K), slowing them to useful energies before distribution to a suite of 22 neutron-science instruments.
Cryogenic requirements
All the superconducting RF cavities in the proton linac operate in saturated 2 K liquid-helium baths.
The supercritical hydrogen moderator absorbs up to 30 kW from the spallation neutrons; the heat is removed by a hydrogen/helium heat exchanger that is in turn cooled by a 15 K supercritical helium flow.
The neutron-science instruments require a maximum of 7500 L/month of liquid helium for cooling of components and sample environments.
The ESS cryomodule test stand, together with a test stand at Uppsala University in Sweden, allow testing of all cryomodules containing RF cavities at full RF power and various operating temperatures (2 K, 4.2 K and 40 K) prior to installation in the linac tunnel.
The test and instruments cryoplant also provides liquid helium to other customers in the Lund region – specifically Lund University and the Max IV synchrotron facility.
Funding and partnership
The ESS is a pan-European project with 13 European nations as members: Czech Republic, Denmark, Estonia, France, Germany, Hungary, Italy, Norway, Poland, Spain, Sweden, Switzerland and the UK.
Significant in-kind contributions of equipment and expertise – from over 40 European partner laboratories – are expected to finance more than a third of the overall construction costs for ESS.
ESS will deliver its first science in 2023, with up to 3000 visiting researchers expected every year once the lab is fully operational.
Joe McEntee is a contributing editor based in South Gloucestershire, UK
from physicsworld.com 16/12/2020
Δεν υπάρχουν σχόλια:
Δημοσίευση σχολίου