Ultrafast photon detectors enable reconstruction-free medical imaging
10 Nov 2021 Tami Freeman
Many medical imaging techniques rely on a mathematical process called tomography, which reconstructs data recorded in one or two dimensions into three-dimensional image volumes. Positron emission tomography (PET) – which images the body using an injected radiotracer that emits positrons as it decays – offers the unique possibility of reconstruction-free 3D imaging. This is because PET can also localize the signal source by exploiting the time difference between detection of the two photons created when the positrons annihilate with electrons in the body.
Today’s state-of-the-art PET systems have a timing resolution of around 210 ps that, based on the speed of light, translates to a spatial localization of 3.15 cm. As such, image reconstruction is still required to create accurate images. But if this timing resolution could be improved, the tomography step could be eliminated completely.
A collaborative team from the University of California, Davis and Hamamatsu Photonics has now developed radiation detectors with an average coincidence timing resolution of 32 ps. The researchers – also from University of Fukui and Kitasato University – have used these detectors to create the first experimental cross-sectional medical image that doesn’t require tomography, a technique they call direct positron emission imaging (dPEI). They report their findings in Nature Photonics.
Ultrafast detection
First author Sun Il Kwon and colleagues achieved dPEI by combining three technology innovations. Firstly, they exploited the Cherenkov luminescence emitted when annihilation photons interact in the detector. If the detector material has a high refractive index and high atomic number, this interaction creates electrons with sufficient energy to produce Cherenkov photons.
To convert this prompt optical signal into an electronic signal, the researchers developed a photosensor based on microchannel plate photomultiplier tubes (MCP-PMTs), which have extremely high single-photon time resolution (SPTR), approaching 20 ps. They integrated the Cherenkov radiator (lead glass) with the photocathode in the MCP-PMT to eliminate any optical boundaries and increase the chances of detecting the small number of Cherenkov photons. Finally, they employed convolutional neural networks (CNNs) to predict the timing information for detected events from the detector waveforms.
To assess the performance of the new detector, the team measured the signal from a 22Na point source placed between two Cherenkov-radiator-integrated MCP-PMTs. The set-up detected Cherenkov photons with an average timing resolution of 32 ps, allowing annihilation events to be localized with a spatial precision of 4.8 mm.
Experimental set-up: The brain phantom in the imaging system. (Courtesy: Sun Il Kwon)

The researchers then tested the system using three test objects filled with the PET radiotracer 18F-FDG: an image quality phantom; a spatial resolution phantom; and a 2D Hoffman brain phantom representing 18F-FDG distribution in a slice of human brain. To capture the signals, the detector pair was translated linearly over the width of each object. The system then created images directly from the measured data, without using any tomographic reconstruction algorithm.
The image quality phantom has a uniform background activity with two 8-mm diameter voids filled with air and with water, all of which could be clearly visualized. The dPEI image of the resolution phantom resolved 3 mm rods, demonstrating a spatial resolution of 4–5 mm. The dPEI image of the larger 18.4-cm diameter brain phantom faithfully captured its detailed structure, with a spatial resolution of around 4.8 mm, suggesting that the method could be scaled for human imaging.
The two-detector set-up, with its average timing resolution of 32 ps, produced images with a similar spatial resolution to that achieved by diagnostic PET scanners. The team note that these initial experiments used long acquisition times and high levels of radioactivity. The signal collection efficiency could be increased, however, by replacing the lead glass radiator in the MCP-PMTs with a higher atomic-number radiator, increasing the radiator thickness, and tiling multichannel detectors to increase geometric coverage.READ MORE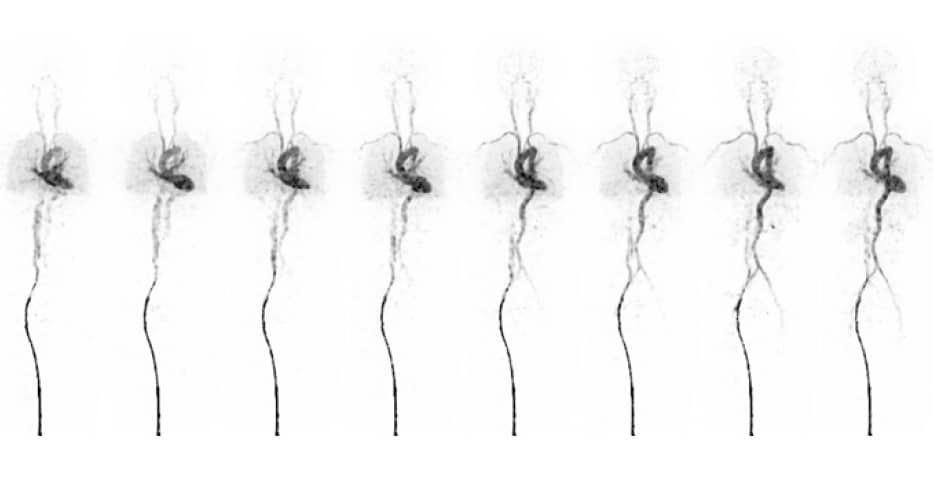
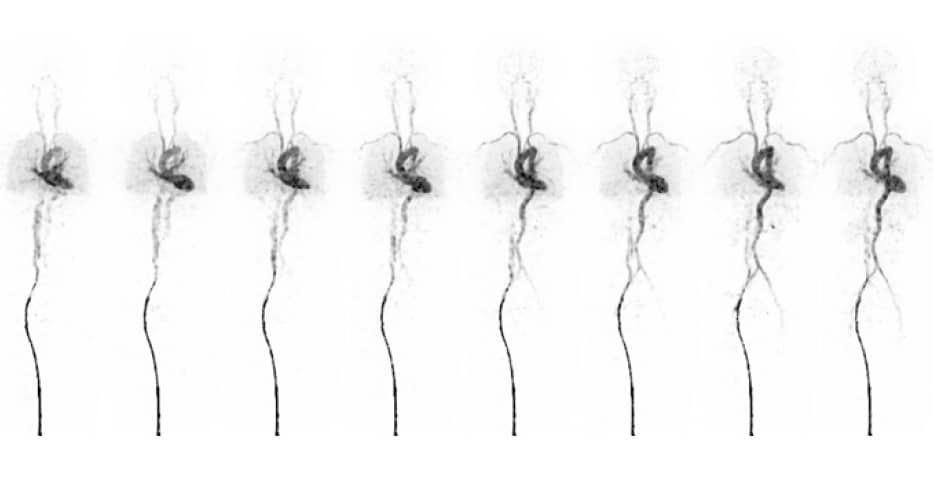
Such an upgraded system would enable shorter acquisition times and/or lower radiation doses. For example, these three changes could reduce the data acquisition time for the brain phantom image from 24 h to roughly 1 min. In addition, multidetector configurations that cover the entire imaging volume-of-interest would remove the need for detector translation and allow dynamic radiotracer imaging.
The team is already working to implement some of those advances. “We are testing new detectors that have the scintillator bismuth germanate (BGO) integrated in the MCP-PMT, rather than lead glass,” senior author Simon Cherry tells Physics World. “This leads to a significant efficiency improvement because BGO has a higher atomic number; it also produces more Cherenkov light because of its higher refractive index.”
from physicsworld.com 10/11/2021
Δεν υπάρχουν σχόλια:
Δημοσίευση σχολίου