Getting physical with the climate crisis
06 Oct 2021 James Dacey
Taken from the October 2021 issue of Physics World. Members of the Institute of Physics can enjoy the full issue via the Physics World app.
With world leaders set to gather in Glasgow next month for the United Nations’ COP26 climate summit, James Dacey examines four vital challenges where physicists can help the world to decarbonize and adapt to the reality of global warming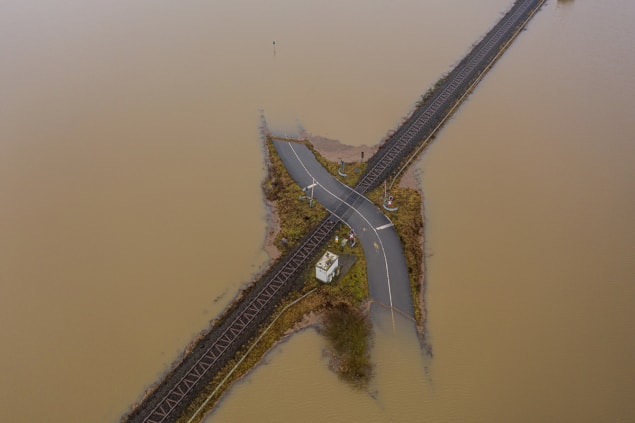
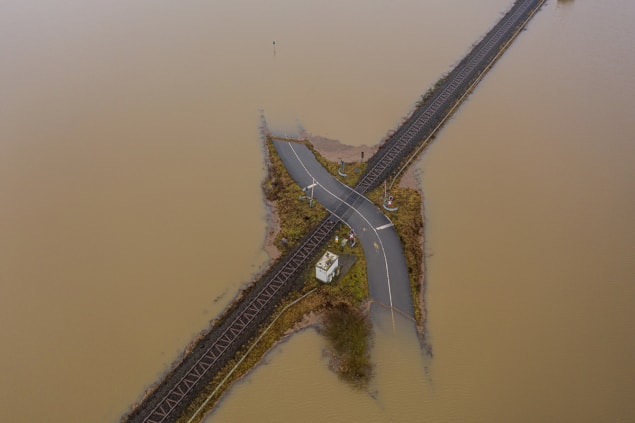
Making pledges is easy. More than 130 nations have so far promised to become “net-zero” carbon emitters by 2050 to try to limit global warming to 2 °C above pre-industrial levels. But as world leaders meet on the banks of the Clyde in Glasgow for the COP26 summit, they will have to turn talk into action. We’ve already hit 1.2 °C warming and there are clear signs of the climate chaos we’re starting to unleash.
The previous summit, held in Madrid in 2019, ended in frustration with little progress on issues such as carbon financing or sharing climate burdens between nations. Politicians floundered despite half a million protestors marching through the Spanish capital. Mainstream journalists seemed more interested in Greta Thunberg’s trans-Atlantic journey to the conference by low-carbon catamaran than in demanding action.
Long term, climate change is a greater threat than the COVID-19 pandemic. It’s also more complicated, affecting lives in clandestine ways, exposing and amplifying global inequalities. The good news is that physicists have a vital part to play, being good at deconstructing problems into their essential elements and speeding up the development of solutions. Basic and applied research will also help to tease out the finer details of the climate system itself.
Here are four key climate questions where physicists are perfectly placed to help.
1 Where will the world’s hot danger zones be?
Thanks to its delightfully warm summers, the resort of Lytton in British Colombia has long been known as “Canada’s hot spot”. Nestled in a valley where the Fraser and Thompson rivers meet, its official website hailed this community of 250 residents as “the perfect place for people looking for the peace and friendliness of a charming small town”. On 29 June, however, tragedy struck when Lytton recorded Canada’s highest ever temperature of 49.6 °C. Wildfire engulfed the town. Two residents were killed and the rest were evacuated as were those in surrounding First Nation communities. Almost all of Lytton’s properties were destroyed.
The unfortunate events were a result of this year’s extraordinary heat wave in western North America, which followed a sequence familiar to meteorologists. An area of high pressure above the Pacific Ocean was driven eastwards through the jet stream by a “Rossby wave” – a planetary-scale fluctuation arising from the Coriolis force. The Rossby wave eventually “broke”, dumping its energy – like an ocean wave hitting the shore – to create an area of high pressure locked over western Canada and US.
Up in flames After recording Canada’s highest ever temperature on 29 June 2021, Lytton in British Columbia had to be evacuated as wildfires engulfed the town and destroyed most of its buildings. (Courtesy: Canadian Press/Shutterstock)
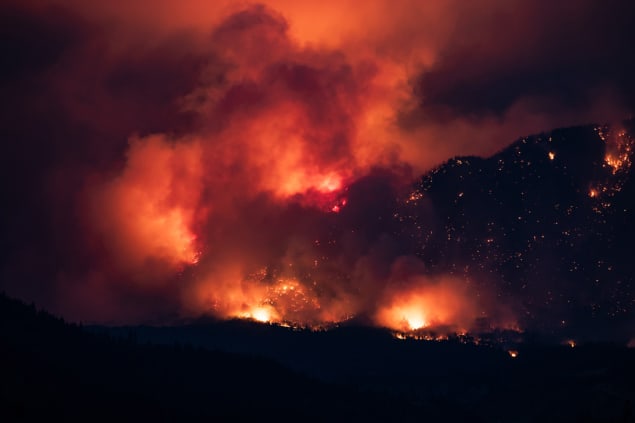
As air sunk through the atmosphere, it got squeezed and heated. Using attribution techniques – an emerging field that blends climate modelling and statistics – an international team of researchers quickly concluded that the daily maximum temperatures in the heatwave region were so extreme that they would normally occur only once every 1000 years. Without human-induced climate change, that heat would be at least 150 times rarer.
And this is where physicists can help by building a more complete understanding of how global warming triggers localized outbreaks of extreme heat. Where are they most likely? How extreme might such occurrences be? And how are they linked to wildfire, air pollution and other hazards? Extreme heat is not just an abstract notion: if we can’t cool our bodies enough, we’re in danger of neurological failure, organ failure and even death, with the risks highest for children and the elderly. Conditions will be particularly bad in regions, such as southern Asia, that are already hot and humid.
How physicists are tackling the climate crisis
Introducing key research questions in climate science and climate solutions
Play Video
To gauge the combined effects of heat and humidity, health experts use a practical unit known as the “wet-bulb temperature”, which is simply the value read by a thermometer wrapped in wet cloth. Spend too long in a wet-bulb temperature above 35 °C and you’ll be in trouble. A 2017 study led by geographer Camilo Mora from the University of Hawaii found that around 30% of people around the world are already exposed to climatic conditions above this deadly threshold for at least 20 days a year (Nature Climate Change 7 501). This figure will increase to nearly half of the world’s population by the end of the century, the authors say, even with drastic reductions in greenhouse-gas emissions.
Limiting warming to 2 °C over pre-industrial levels could avoid thousands of heat-related deaths compared with the 3 °C rise we’re heading towards Eunice Lo, University of Bristol
“One key challenge is to quantify the different combinations of heat and humidity, then to link them with different health outcomes,” says Eunice Lo, a physicist-turned-climate scientist at the University of Bristol, UK. By combining historical health data (1987–2000) in 15 US cities with a statistical model of temperature-mortality, her group has found that limiting warming to 2 °C over pre-industrial levels could avoid thousands of heat-related deaths compared with the 3 °C rise we’re heading towards by the end of the century based on current emissions pathways (Science Advances 5 eaau4373).
Particularly important will be to identify hotspots where dangerous heat and vulnerable populations coincide. City dwellers are at most risk due to heat being reflected and absorbed from buildings, roads and other urban structures. This “urban heat island” creates zones within cities that can be several degrees warmer than the surrounding countryside. Recent studies suggest that it disproportionately affects communities along both economic (Env. Res. Lett. 14 105003) and racial lines (Nature Comms 12 2721).
2 How can we track and reduce the hidden carbon in buildings?
Can a building be evil? That was the provocative question posed by David Coley, a physicist at the University of Bath, UK, to an audience at the headquarters of the Institute of Physics (IOP) shortly before it relocated to its environmentally sound premises at King’s Cross, London, in 2018. Coley, who studies energy use in buildings, was pointing out that when we evaluate architecture, we must always consider its environmental harm, not just its beauty or functionality.
Fortunately, architects are listening to environmental concerns, with sustainability one of the 21st century’s key themes in building design. Companies and organizations (including the IOP) are not shy in boasting about the green credentials of their premises, from green roofs and ground-source heat pumps to smart lighting and renewable energy. What’s harder is knowing the carbon footprint of building materials throughout their lifecycle, which requires reams of data about supply chains and logistics that either don’t exist or have never been tracked.
Unfortunately, unless politicians can agree on a global carbon price and make businesses pay for, or trade, carbon emissions – an issue on the agenda at COP26 – there will be little incentive for construction companies to keep detailed records. “In most cities it is a challenge to even know how many buildings there are, how many kilometres of road, how many miles of water pipes there are,” says Arpad Horvath, an environmental engineer at the University of California, Berkeley, and co-chair of IOP Publishing’s Environmental Research 2021 virtual conference.
Horvath is often hired by businesses to help make their buildings more green, but says that when he asks what they are made of he just gets a shrug of the shoulders before being invited to stroll around and figure it out himself. In the coming years, better data collection, along with tools such as smart sensors and 3D-mapping drones, should create a more accurate picture of carbon embedded within urban infrastructures. Learning from the past will also be essential to develop more sustainable building practices.
In particular, we’ll have to readdress our love affair with concrete. It’s a great building material because it’s cheap and resistant to rust, fire and biological pests. Trouble is, its key constituent is cement, which is made by converting limestone (calcium carbonate) into lime (calcium oxide) in a process that spews out roughly a tonne of CO2 for every tonne of cement. Overall, cement accounts for 3% of the world’s carbon emissions.
Wood you believe? Mjøstårnet in Norway (top), designed by Voll Architects, is the tallest wooden building in the world. The football club Forest Green Rovers has commissioned an all-wood design from Zaha Hadid Architects (bottom) for its new stadium in Stroud, UK. (Courtesy: Shutterstock/Kristin Spalder; Zaha Hadid Architects)

Annual global cement production has remained stuck at just over 4 × 109 tonnes over the last decade and ditching concrete is unlikely anytime soon. In his book Making the Modern World, the Czech-Canadian energy researcher Vaclav Smil estimates that China used more cement between 2011 and 2013 than the US did in the entire 20th century. What’s worse is that concrete is often reinforced with steel, the manufacture of which also releases CO2.
Physicists can help by devising cleaner ways of producing cement and steel. But developing alternative building materials will be vital too. Among the promising options are timber, rubber, coconut fibres, bamboo and other “biogenic” materials, all of which have a lower carbon footprint and act as a carbon sink. Large timber buildings are now becoming fashionable, the tallest being an 18-storey, 85 m tower in Brumunddal, Norway. One English football club – Forest Green Rovers – is even planning the world’s first entirely wooden sports stadium.
3 How will clouds influence future climates?
Do you see dolphins, dogs or dinosaurs when you stare at the clouds? You can be forgiven for seeing all sorts of strange shapes as clouds are complex, varying and always changing. Even a seemingly featureless, low-lying stratus cloud is, to meteorologists, a dynamic system with varying temperatures, humidities and wind speeds. In terms of the climate, clouds create a complicated duality: they not only reflect the Sun’s radiation (a cooling effect) but also trap infrared radiation emitted from the Earth’s surface (a warming effect).
On balance, clouds nearer the stratosphere warm us, whereas low-lying clouds tend to cool us because their greenhouse effect is smaller. Taken together, however, clouds in today’s atmosphere have an overall net cooling effect. The crucial question, though, is how clouds form; the key step being water vapour condensing around dust, salt and other aerosol particles to form droplets. When the air cools to its dew point it cannot hold as much water vapour, and it is these isolated droplets that conglomerate into clouds.
Clouds form a very complicated part of the Earth’s climate, having both cooling and warming effects. Understanding cloud formation is key to improved climate models. (Courtesy: iStock/imagedepotpro)
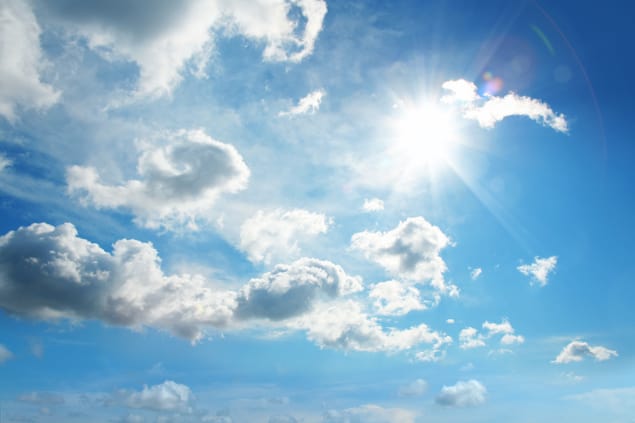
The science gets murkier when linking individual cloud formation with global climate models. “There’s such a vast range of scales we have to deal with – from a planet to a dust speck – and you can’t do all of that at once,” says Steven Sherwood, an atmospheric physicist at the University of New South Wales (UNSW) in Australia, who uses numerical simulations of cloud formation to test assumptions built into global climate models.
Cloud models need to be tested against real-world measurements. Satellites have been tracking clouds for the past four decades, and for 20 years LiDAR has been capturing high-resolution 3D information about cloud dimensions and water content. Experimentalists on the CLOUD project at CERN have even been using cloud chambers to examine the role of cosmic rays in creating aerosols in the atmosphere.
We know that as the average global temperature increases, the greenhouse effect from clouds will increasingly outweigh their reflective cooling powers. By how much, though, is unclear
We know that as the average global temperature increases, the greenhouse effect from clouds will increasingly outweigh their reflective cooling powers. By how much, though, is unclear. Another confusion is that climate models predict that warming will be more pronounced at higher latitudes – but not by as much as in past warm global climates with similarly high greenhouse-gas concentrations.
“One explanation is that we don’t understand some of the cloud processes in settings where temperatures are warmer, or when there were much higher methane concentrations in the atmosphere,” says physicist Katrin Meissner, who is director of the USNW’s Climate Change Research and another co-chair of IOP Publishing’s conference. Whatever the reason, understanding the impact of changing cloud cover will be vital.
Global warming of more than 2 °C above pre-industrial levels will make countries at higher latitudes cloudier, wetter and more likely to suffer from flooding. The extreme flooding that resulted in at least 200 fatalities, the majority in Germany and Belgium (see photo at top of article) could be a grim marker of things to come. Most subtropical regions, in contrast, will become drier, increasing risks of drought and wildfire in the Mediterranean, Middle East and Australia. As for the tropics – where rain is vital for food security – we are even less certain.
4 Can we safely store enough carbon underground?
Extracting CO2 from industrial plants and pumping it underground might not have the green glamour of an elegantly rotating wind turbine. But if deployed at scale, carbon capture and storage (CCS) technology could be crucial in reaching net-zero global emissions by 2050. The basic principles are almost a century old, and the first commercial CCS project designed specifically to combat climate change – the Sleipner project off the Norwegian coast – opened in 1996. It’s been sequestering a million tonnes of CO2 annually ever since.
Capturing CO2 directly from the air isn’t easy, as its concentration there is so low. That’s why many commercial CCS efforts instead extract it directly from the exhaust gases at power plants. The gas is usually compressed into a supercritical fluid state and then pumped into porous rocks covered with impermeable “caprocks” that form a natural seal. Geologists have identified plenty of suitable storage sites around the world and most climate-mitigation scenarios that limit warming to 2 °C above pre-industrial levels include a lot of CCS.
But despite Sleipner’s success, there are just 26 commercial CCS facilities currently in operation, which together store barely a few million tonnes of CO2 from the 35 billion tonnes emitted each year. It’s an energy-intensive process, with chemical solvents and heat usually separating the CO2 from flue gases, and energy needed to liquefy the CO2, transport it and pump it into rocks. Then there are the ongoing costs of building and maintaining infrastructure.
For CCS to be used more widely, it must become more energy efficient and economically viable. To improve the capture process, researchers will need to develop better chemical processes or design nanoscale membranes to filter the gas instead. On the storage side, one option is to reuse redundant infrastructure from the oil and gas industry in well-surveyed geological settings. That at least is the aim of the Acorn project, which would be the UK’s first commercial CCS plant when it opens in the mid-2020s. Based at the St Fergus gas terminal in north-east Scotland, it will repurpose North Sea gas pipelines to transport CO2 to a site roughly 80 km from the coast, where it will be pumped into sandstone lying underneath shale.
Avoiding leaks will be vital too, although supporters of CCS insist there are fewer risks of that happening over time as CO2 gradually dissolves, sinks and then gets trapped in pores before eventually precipitating out as carbonate minerals. “CCS contrasts very much with nuclear waste storage, where over time we know there are going to be problems,” says Martin Blunt, an engineering physicist at Imperial College London. “CO2 storage is not like that.”
But industrial negligence does happen. And can we be sure the CO2 won’t gradually seep to the surface over millennia? Fortunately, when researchers from the universities of Aberdeen, Barcelona and Edinburgh modelled leakage rates in different scenarios, they found that well-regulated storage should keep leakage below 0.0008% per year, with over 98% of the injected CO2 stored over 10,000 years (Nature Comms 9 2201). Even with multiple abandoned wells, more than 78% would be retained, the study found.
Disappearing act Run by Reykjavik Energy, the CarbFix project takes gases from Iceland’s Hellisheiði geothermal power plant and stores them in basalt rocks 400 m underground. The company also has pilot projects to apply CarbFix to steel, iron and cement production. (Courtesy: CarbFix)
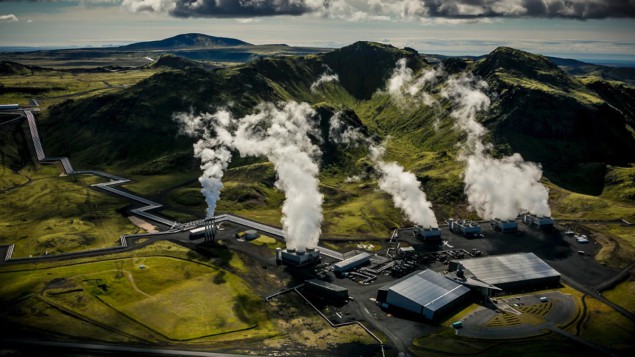
Juan Alcalde Martín, a geoscientist at the Geo3bcn research lab in Barcelona, who led the study, thinks that geophysicists can help by characterizing saline aquifers and other less surveyed rock formations that could also be used for CCS. One novel approach is being taken by Reykjavik Energy, an Icelandic company whose CarbFix project involves injecting CO2 into basalts. These rocks mineralize the gas – essentially turning it into stone – much faster than sedimentary rocks can. And although the process uses a lot of water, the beauty of basalt is that it can be found below most of the world’s oceans.
But no matter how enticing individual solutions appear, adapting to live with our changing climate will require a mix of actions, with physicists playing a key part. “I encourage everybody to join the movement to make the world more sustainable,” says Horvath at Berkeley. “It might have been a slogan 30 years ago; it might have even been a slogan 10 years ago. Now it’s a necessity.”
IOP Publishing’s Environmental Research 2021 virtual conference is free to attend and runs from 15 to 19 November.
from physicsworld.com 3/11/2021
Δεν υπάρχουν σχόλια:
Δημοσίευση σχολίου