Let’s talk about quantum 2.0: why we need to sharpen up our language
24 Apr 2023 Robert P Crease
Quantum technology could benefit from us finding less spooky ways to describe the weird phenomena on which they’re based, argue Robert P Crease, Jennifer Carter and Gino Elia
Words matter Our inability to find the right language to describe quantum phenomena could be holding back the development of quantum technology. (Courtesy: iStock/Anadmist)
Superposition, entanglement and other baffling facets of the quantum world are now the driving forces behind various breakthrough technologies. Whereas “quantum 1.0” was all about interrogating the mysteries of Schrödinger’s wave equations and setting up clever experiments to close loopholes in the theory, “quantum 2.0” is putting the most bizarre aspects of quantum physics to routine work. Quantum computers based on superposition, as well as encryption devices relying on entanglement for long-distance communication, are now all becoming technologically viable.
But despite the burgeoning growth of quantum technology, one thing that hasn’t changed is the cumbersome and counterintuitive language we use to talk about all things quantum. While the reality of entanglement and superposition is beyond all reasonable doubt, it is as maddening as ever to describe them in words. Quantum phenomena are strange, but that does not mean we should be satisfied with strange language to describe them.
From the very early days of quantum mechanics, Albert Einstein, Niels Bohr, Werner Heisenberg and others strove to understand this new-fangled non-classical physics of quantum 1.0. Their struggle concerned a gap between how we talk about phenomena and how we encounter them in the laboratory. That gap was created by the imperfect metaphorical language still largely used to characterize non-classical phenomena.
While the reality of entanglement and superposition is beyond all reasonable doubt, it is as maddening as ever to describe them using words
The concept of “entanglement” can’t help but evoke two (or more) discrete things being woven together yet somehow also separate, like tangled skeins of yarn. As for “superposition”, it conjures up the image of a cloud of different states just before some external cause selects one state, while the others vanish. Or think of terms and phrases like “field”, “path”, “self-interference”, “collapse of a wave function” or a “photon choosing to go back in time”. There’s a big gap between what’s being pictured and the phenomena they label.
Language counts
Physicists usually have a firm enough intuitive grip on what’s happening when immersed in their craft that they’re generally not that bothered by these terms, even if sometimes they’re still a mystery. In quantum 2.0, however, with its soon-to-be-commonplace devices and future applications, we should be careful how we use the language we inherited from quantum 1.0. There are two reasons why.
The first is clarity. If scientists can’t straightforwardly describe how these devices and applications work, it makes the devices seem mysterious and other-worldly. Spooky and counterintuitive language also makes scientists seem like priests, anointed individuals who connect with the beyond. If physicists can’t put things in language that others understand, it implies that no language makes sense, or physicists can’t find one that does, or they’re making things up. This ultimately encourages scepticism and science denial, as well as acceptance of scientific illiteracy.
A second reason is practical. Finding the right language for quantum effects can help avoid confusion in developing quantum 2.0 technologies. Bad metaphors may make certain kinds of devices – quantum telephones, human teleportation devices – seem more physically plausible than they are. On the other hand, taking metaphors too literally – hewing too closely to the pictures conjured by them – can tilt the thinking of designers in the wrong direction. Better pictures of the real will help to plan better experiments to study it.READ MORE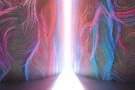
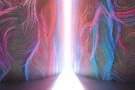
The word “entanglement”, for instance, is a good way of talking about quantum physics in certain areas when we can cast behaviour in terms of particles. But we can’t think of discrete energy states in a field too literally as particle-like; that is, independent of each other. To do so would require a mechanism for their dependence. That, in turn, would need other metaphors, such as the wave function being able to “choose” its states, which in turn demands either non-local effects or superluminal communication.
As for “superposition”, it is also a metaphor that works in certain situations, such as those where it seems as though possibilities exist simultaneously. But this suggests there is a kind of “container of possibilities” – like an electron in a potential well – that shows up only at the quantum scale. This, in turn, implies that quantum and classical phenomena are separated by a distinct border rather than by a difference of degree. The metaphor is therefore hard to apply to, say, macromolecules, quantum liquids or the quantum fluctuations near the event horizon of a black hole, where the two bleed into each other.
The critical point
Bohr famously held that we cannot make a literal picture of quantum phenomena, which poses a seemingly insurmountable obstacle to precise language. But he did not mean we should abandon the attempt to create language that we really and truly understand that describes accurately what we encounter. Bohr struggled mightily to create a language that reconciles the peculiarity of quantum phenomena with the ordinary language used to describe experimental situations. Still, there is no reason to think that it is impossible to develop a language that successfully describes quantum phenomena.
There is no reason to think that it is impossible to develop a language that successfully describes quantum phenomena
QBism is one attempt. QBist language combines the resources of Bayesian probability and quantum information theory to treat the preparation of quantum systems, not as picking out wave-like or particle-like things, but drafting a probabilistic assessment of measurement outcomes for the user. Instead of seeing, say, a photon with unknown polarization as “making a choice” about its polarization when shot through a calcite crystal, the QBist approach treats the result as “updates” in our “information about the system”.
This language provides a unified description, but does not insist that the photon is “particle-like” or “wave-like”. Not all physicists are satisfied with QBism, and it might not be the only such approach to characterizing quantum phenomena. But any alternative to QBism will have to help us see what’s really puzzling about quantum mechanics without us getting stuck on past characterizations of the puzzles. If such an attempt succeeds, we’re truly on the threshold of quantum 2.0.
Robert P Crease (click link below for full bio) is chair of the Department of Philosophy, Stony Brook University, US. Jennifer Carter is a lecturer in philosophy at Stony Brook, where Gino Elia is a PhD student

Robert P Crease is chair of the Department of Philosophy, Stony Brook University, US and writes a monthly column for Physics World, robertpcrease.com, e-mail robert.crease@stonybrook.edu. His latest book is The Leak: Politics, Activists, and Loss of Trust at Brookhaven National Laboratory (MIT Press)
FROM PHYSICSWORLD.COM 29/4/2023
Δεν υπάρχουν σχόλια:
Δημοσίευση σχολίου