Simulations shed light on mechanisms of DNA damage during proton therapy
13 Apr 2023 Rojin Jafari
Damage simulations Changes in electron density when a proton passes through the centre of solvated DNA. Red indicates increases in electron density, blue indicates decreases. (Courtesy: Chris Shepard)
Understanding the radiobiological response of DNA to charged particle irradiation is crucial to help optimize particle therapies and improve radiation protection strategies. DNA damage in extreme conditions (such as those experienced by astronauts, for example) can result in double-strand breaks, which can lead to mutations, chromosomal aberrations and changes in gene expression.
Interactions between charged particles and the electronic structure of DNA are complex, however, and there is a significant gap in understanding the dependence of different types of DNA damage on high-energy protons. Towards filling this knowledge void, researchers at the University of North Carolina at Chapel Hill and Massachusetts Institute of Technology used large-scale computer simulations to identify a mechanism through which ionizing radiation could cause a break in one or both of the DNA strands. Their findings, reported in Physical Review Letters, could help improve proton therapy for cancer treatments and enable better protection during human spaceflight.
Christopher Shepard and colleagues employed real-time time-dependent density functional theory (TD-DFT) simulations on a supercomputer. TD-DFT is a method that computes the electron density of a many-electron system using a single function. Therefore, it is considered to be an efficient method for calculating the electronic structure of large systems.
With this in mind, the researchers used TD-DFT to quantify at the molecular level the energy transfer from high-energy protons to solvated DNA (DNA surrounded by water), a state in which the DNA is separated into its sugar-phosphate side chains and nucleobase backbone components.
DFT calculations account for large-scale interactions between the electrons, using a set of approximations that make it possible to compute the electronic structure of complex DNA when exposed to ionizing radiation and study the time-dependent electron density.
The computational approach
For their computations, the researchers focused on the total energy of the solvated DNA system. In TD-DFT, total energy is a function of the electron density, and by moving the proton at a constant velocity, the change in total energy can be used to measure the electronic energy transfer.
The researchers studied two different proton paths, with each path closer to a different region of DNA: either the sugar-phosphate side chains or the nucleobases. They found that spread change (a measure of electronic delocalization) of DNA occurs close to the path of the irradiating proton and is higher in trajectories closer to the phosphate chains. This means that the DNA’s sugar-phosphate side chain molecules absorbed much more energy than did the nucleobases.
More precisely, the simulations showed that the amount of energy transferred to the solvated DNA system by an incoming high-energy proton depends on the part of DNA component that it comes closest to. The researchers determined that the energy transferred to the sugar-phosphate side chain is more than two to three times larger than the energy transferred to the nucleobase when a proton is equally close to either of the two components. Consequently, side chain irradiation is more likely to result in damage.READ MORE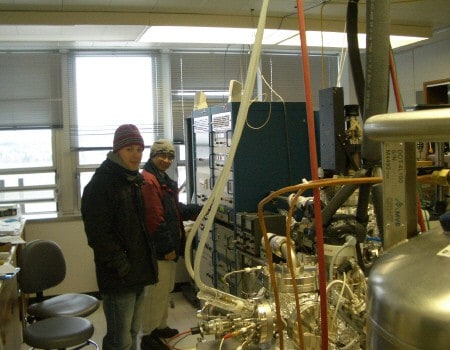
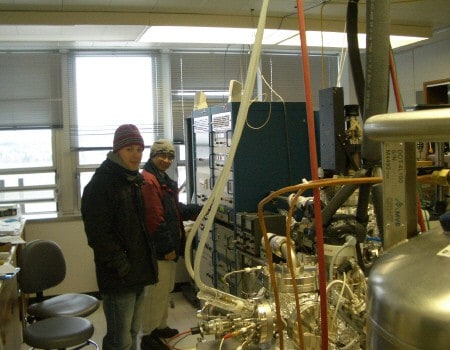
The findings indicate that there is a higher prevalence of high-energy electron–hole formation in the sugar-phosphate side chains, which in turn, can lead to formation of free radicals. Free radicals, aqueous atoms or molecules with an unpaired valence electron, are highly reactive with their local surroundings and, as a result, can be highly damaging. Therefore, interactions between the sugar-phosphate side chains and generated radicals can lead to fractures and eventually break one or more DNA strands.
According to an accompanying Viewpoint article, this work provides an advance towards studying complex interaction dynamics that are difficult to replicate in a laboratory setting. Some caution should be exercised, however, until detailed experiments test the researchers’ models and findings.
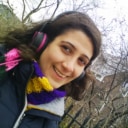
Rojin Jafari is a PhD student contributor to Physics World working in soft condensed-matter physics at Johns Hopkins University. Find out more about our student contributor networks
FROM PHYSICSWORLD.COM 17/4/2023
Δεν υπάρχουν σχόλια:
Δημοσίευση σχολίου