Quantum computing could tackle radiotherapy’s intractable problems
10 Aug 2023 Tami Freeman
Attendees at the recent AAPM Annual Meeting learnt how quantum computers could potentially be employed to enhance radiation therapy
Processing power Radiation oncology could exploit two key properties of quantum computers: their vast storage capabilities and their ability to perform operations on multiple datasets simultaneously. (Courtesy: Shutterstock/Bartlomiej K Wroblewski)
Quantum computing is a rapidly evolving technology offering the promise of exponentially greater computing power than that achievable by today’s classical computers. So could the field of medical physics exploit these emerging systems, and how best could such immense processing power be harnessed for clinical benefit?
Speaking at the recent AAPM Annual Meeting in Houston, TX, Amit Sawant from the University of Maryland School of Medicine examined the potential applications of quantum computing within radiation therapy. “Radiation oncology as a discipline is probably more power hungry than most. We have large, spatially and temporally rich datasets, and we also have very intricate clinical workflows,” he explained.
Looking at advances in traditional computing technologies, starting from the first general-purpose digital computer built in 1945, Sawant noted that “every three or four decades we have pretty much increased our computational power by six orders of magnitude”. Quantum computers, however, have potential to massively surpass that progress by producing an exponentially higher increase. “That’s humbling and really expands our thinking,” he told the audience.
Appropriate applications
Classical computers store information in “bits”, whereas quantum computers use quantum bits, or “qubits”. These qubits are based on a physical system that can be in either of two quantum states (such as the up and down spin states of an electron, for example, or the horizontal and vertical polarization of a photon). The big difference is that a while a bit can be either 0 or 1, qubits can also exist in a superposition of the two states. This results in a far greater storage capacity – with the amount of data that can be stored increasing exponentially with the number of qubits – and an equivalent increase in processing power.
Within radiotherapy, access to higher speed computing could help in many day-to-day clinical tasks, such as contouring, image reconstruction, plan optimization and dose calculations, Sawant explained. But he pointed out that conventional computing will improve to meet these needs, with 10- or 100-times faster computers likely emerging over the next few years. “This is not really what quantum computing needs to be harnessed for,” he said.
Instead, he suggested, radiation oncology should exploit two key properties of quantum computers: their vast storage capabilities and, once large amounts of data are stored, their ability to perform operations on all these data simultaneously. “These two properties make quantum computing ideal for radiotherapy optimization, because we have lots of variables on which we need to run the same operation to find the global minimum.”
Sawant explained that radiotherapy optimization is a computationally hard and complex problem, with various assumptions and approximations employed to create an optimized plan in a clinically viable time. But as delivery techniques become increasingly complex – using non-coplanar beams or multiple arcs, for instance – this approach doesn’t always guarantee an optimum result. Quantum computers could remove the need for approximations and guarantee that global minima are reached, he said.
He described an example of such a complex optimization: real-time volumetric motion management for radiotherapy of moving tumours. “We should not deliver radiation by just chasing the target, we should be chasing the entire volume because it is all affected by radiation,” he explained. Including surrounding normal tissue into the optimization process, however, makes this a huge problem. “We use a combination of particle swarm optimization and mixed integer programming to achieve this, which gives dosimetric benefits, but is not practical in the clinical environment.”
Ventilation map Functionally weighted airway sparing aims to preserve lung function after treatment. (Courtesy: Phys. Med. Biol. 10.1088/1361-6560/ab9f5d)

Sawant and his team are also investigating functional lung sparing, in which a radiotherapy plan is created that ensures ventilation is preserved after treatment. This is another highly complex problem – with each airway segment in the lung designated as an organ-at-risk (OAR), instead of the usual 10 or 20 OARs, there are now 250 to consider. Again, this can be achieved using swarm optimization techniques on very high-end computers, but the process still takes several hours to several days.
“These problems definitely can be tackled by quantum computers,” said Sawant. “But that’s still thinking small. What if you had a million or a billion times higher computational power, what could you do then?”
Future gazing
One limitation of current radiotherapy is its anatomy-focused approach, in which each organ is treated independently. The human body, however, contains many interacting systems – the cardiovascular system, the respiratory system and so on – that really should be considered together. For example, Sawant explained, if radiotherapy affects the lungs then the heart gets stressed, and when the heart is stressed the lungs have to work faster to produce the same output. But currently, treatment plans aren’t optimized to preserve function in the two organs simultaneously.
Digital twin Multiscale models of the human body that span multiple time and length scales could help predict treatment response. (Courtesy: CC BY 3.0/BioMed Res. Int. 10.1155/2014/902545)
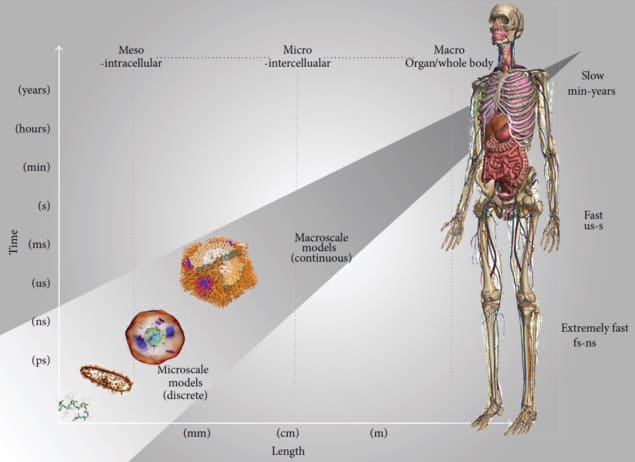
“The next step is to look at all of the human body as a set of interconnected systems – we cannot even visualize doing that right now because we don’t have the computational power,” said Sawant. “The optimization problem is becoming more and more exponential in its scope. Quantum computing may help us solve these large problems.”
Looking further into the future, Sawant proposed the idea of creating a radiotherapy-specific digital twin of a human. This would be a multiscale model, ranging from the cellular level to the anatomic and system levels, that should more accurately predict a patient’s response to radiotherapy. It could also enable optimization over multimodal treatments, including chemotherapy, immunotherapy and surgery. “This is a problem that’s worthy of such high computational power,” he said.
“Quantum computing represents intriguing possibilities for oncology. It forces us to think bigger than we have ever before,” said Sawant. He emphasized that this is not an immediate solution, as it will require the development of very different algorithms and data structures to fully exploit its potential. “But overall, I think the future is very exciting,” he concluded.READ MORE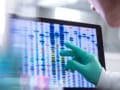
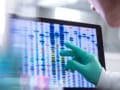
As for the likely first role of quantum computing in radiation oncology, Larry Antonuk from the University of Michigan, who organized this AAPM session and presented the introductory talk, suggests that treatment planning may be first to benefit.
“Considering the challenges facing the ambitious goals for quantum processor development and quantum error correction, it’s likely we’ll first see rudimentary (followed by increasingly sophisticated) efforts to harness the growing capabilities of quantum computing to improve the quality of treatment plans,” Antonuk tells Physics World. “This will eventually be followed by efforts to provide real-time improvement to the precise delivery of radiation therapy beams.”

Tami Freeman is an online editor for Physics World
from physicsworld.com 11/8/2023
Δεν υπάρχουν σχόλια:
Δημοσίευση σχολίου