Double dose of quantum weirdness pushes sensors past the limit
17 Nov 2022
Extra spooky: Artist's impression of entangled and delocalized atoms within the interferometer. (Courtesy: Steven Burrows, Thompson group/JILA)
For most people, quantum mechanics seems pretty weird. Take the principle of delocalization, which states that a quantum particle can, in some sense, exist simultaneously in multiple locations. Then there’s entanglement: the invisible connection between particles that allows the state of one particle to determine that of another, even across vast distances. But as weird as delocalization and entanglement are, they can be very useful, and physicists at JILA in Boulder, Colorado, US have now incorporated both into a single quantum sensor for the first time. The new sensor can detect accelerations below the usual limit set by noise arising from quantum fluctuations, providing a sharper tool for exploring fundamental physics as well as for applications such as navigation and Earth monitoring.
The JILA team’s experimental setup uses a matter-wave interferometer, which interferes massive quantum particles in the same way as an ordinary interferometer interferes beams of light. While light-based interferometers can be very sensitive – they are used to detect gravitational waves – their matter-wave equivalents can in principle detect even smaller accelerations because the quantum wavelength of massive particles is so much shorter. These sensors therefore offer a way to search for phenomena such as dark matter and dark energy that currently cannot be detected directly, but that nevertheless make their presence known through gravitational effects.
Turn down the noise
In the experiment, atoms are first placed inside an optical cavity, which is a set of opposing mirrors with light trapped in between. The light that bounces between these mirrors interacts with the atoms, causing nearly 1000 of them to become entangled with each other.
The maximum sensitivity of quantum sensors is generally limited by noise produced by the random collapse of individual atoms’ quantum states whenever they are measured. Previous experiments sought to reduce this quantum noise by running the experiment in parallel a multitude of times with many atoms, then averaging out the quantum noise of each individual atom.
In the JILA team’s experiment, however, the researchers tested two alternatives in which the atoms actually conspire with each other to cancel each other’s quantum noise. The first approach involved so-called quantum non-demolition measurements, in which the researchers made a pre-measurement of the quantum noise associated with the atoms and then subtracted this quantum noise from the final measurement. In the second method, the researchers introduced light into the cavity that triggers an atomic process known as one-axis twisting, which causes the entangled atoms in different motional states (or momentum states) to have a lower uncertainty than they would if the atoms were not entangled.
The resulting quantum states are called squeezed spin states because they consist of two levels of momentum states that are essentially “squeezed” together to form an effective spin system. In the JILA team’s experiment, these squeezed spin states allow any quantum phase that accrues between the momentum states due to accelerations to be measured with a higher precision. In both approaches, due to the entanglement between the atoms, the quantum noise becomes correlated between atoms such that one atom’s quantum noise is cancelled by that of another, making the quantum sensor “quieter” and therefore more precise.
All over the place
In the experiment’s second step, the researchers introduced delocalization. Lasers separate the wave packets of the atoms, thereby bringing them into a superposition of different momentum states; as the two parts of the wave packets move apart, each atom is essentially in two locations at the same time. By cancelling this superposition with another laser, the atoms’ wave packets interfere with each other, and any influence on their location – for example due to falling under gravity – can then be detected with ultrahigh sensitivity. Combining this interference experiment with the entanglement approaches made it possible for the researchers to detect accelerations smaller than the standard quantum limit set by the quantum noise of the individual atoms.
James K Thompson, who led the team’s research together with PhD students Chengyi Luo and Graham Greve, says that using squeezed states for quantum sensing is often called “quantum 2.0”, a version of quantum sensing that moves beyond single-particle physics. He mentions that there is “a wonderful synergy” emerging between advances in quantum sensing and quantum simulation, and he is interested in exploiting this in two ways: by using momentum-state encoding of quantum states to perform quantum simulation, and by applying the tools of quantum sensing to measure the evolution of the quantum many-body system. “What we learn can then be used to enhance quantum sensors even further,” he says.READ MORE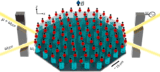
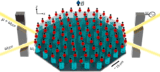
Timothy Kovachy, a physicist from Northwestern University in the US who was not involved in the research, says that the standard quantum limit can be a significant constraint on the precision of quantum sensors. He therefore describes the result as a major step forward for quantum sensing. He says that the incorporation of spin squeezing to surpass the standard quantum limit is essential for these interferometers to reach their full potential and that it is a significant achievement to realize cavity-based squeezing together with intracavity atom interferometry. He adds that cavities can also provide valuable features for atom interferometry, such as improved spatial mode quality and power build-up.
The research is published in Nature.
Martijn Boerkamp is a science writer based in the Netherlands
from physicsworld.com 29/12/2022
Δεν υπάρχουν σχόλια:
Δημοσίευση σχολίου