Liquid metal experiment sheds light on solar corona conundrum
10 Feb 2022 Isabelle Dumé
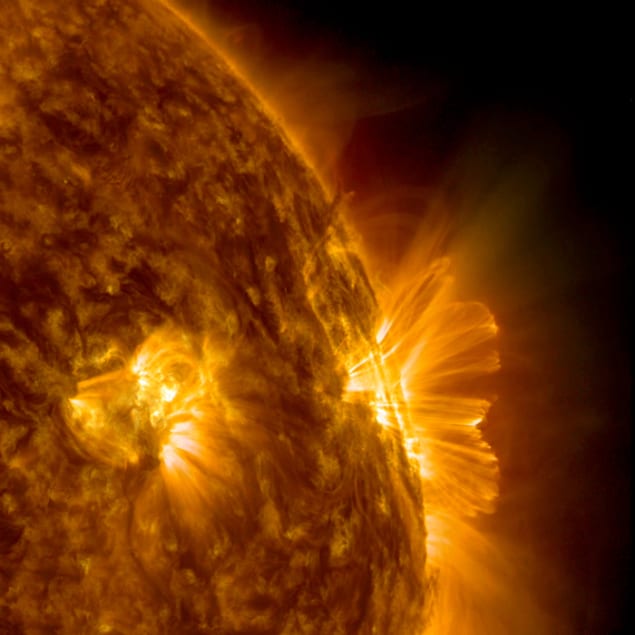
A plasma ejection during a solar flare. (Courtesy: NASA/SDO and the AIA, EVE, and HMI science teams)
The centre of the Sun is incredibly hot, at 15 million degrees Celsius. Its surface is much cooler, at 6000 degrees. Then, in the outer reaches of its atmosphere, it becomes hotter again, with temperatures in the solar corona reaching several million degrees. This “corona heating”, as it is known, is one of greatest mysteries of solar physics. Current theories suggest that it stems from a “hot trail” that forms in the region just below the corona, where strong magnetic fields mean that plasma waves known as Alfvén waves can travel at the speed of sound. Direct evidence for this behaviour has, however, been lacking.
Researchers from the HZDR in Germany have now shed new light on this mystery by confirming the behaviour of Alfvén waves at high magnetic fields for the first time. Using the HZDR’s Dresden High Magnetic Field Laboratory, they showed that the speed of Alfvén waves within a sample of molten rubidium metal can indeed surpass the speed of sound, thus validating a key prediction from the “hot trail” theory.
Magnetism and Alfvén waves
Researchers have known for a long time that magnetic fields must be the main culprit in corona heating. The tricky part is determining whether the heating stems from sudden changes in magnetic field structures in the hot solar plasma just below the corona, or from the dampening of different types of waves.
These different types of waves include Alfvén waves, which were discovered in 1942 by the Swedish plasma physicist Hannes Alfvén. They were initially studied in experiments involving liquid metal, and later in plasma-physics facilities, where they are used to heat plasmas in fusion experiments. In an astrophysical context, Alfvén waves are found in the Earth’s ionosphere, and are thought to play a crucial role not only in corona heating, but also in accelerating the solar wind, which is the stream of charged particles expelled by the Sun.
Reaching the “magic” point
In the latest research, Frank Stefani of the HZDR Institute of Fluid Dynamics and colleagues focused on Alfvén waves that occur in the magnetic canopy, which is a plasma-filled layer of the solar atmosphere just below the corona. Within this canopy, the Sun’s magnetic field runs mostly parallel to the solar surface, and it interacts with ionized particles in the plasma. As the strength of the field increases, so do the frequency and propagation speed of the Alfvén waves. Eventually, if the field becomes strong enough, Stefani explains that sound waves and Alfvén will have roughly the same speed and can therefore “morph” into each other.
To find out what happens at this “magic” point, the HZDR team set out to explore it in an analogous laboratory experiment involving liquid rubidium metal. This is the first time such an experiment had been carried out in high pulsed magnetic fields, and performing it meant overcoming several challenges.
Thanks to previous studies on liquid metals, the team knew that rubidium must reach the magic point at a magnetic field strength of 54 T. This is well below the Dresden facility’s maximum of nearly 100 T. However, because rubidium metal ignites spontaneously in air and reacts violently with water, the researchers had to encase it in a robust stainless-steel container before they could inject the alternating current needed to generate Alfvén waves into the bottom of the container while simultaneously exposing it to the magnetic field.READ MORE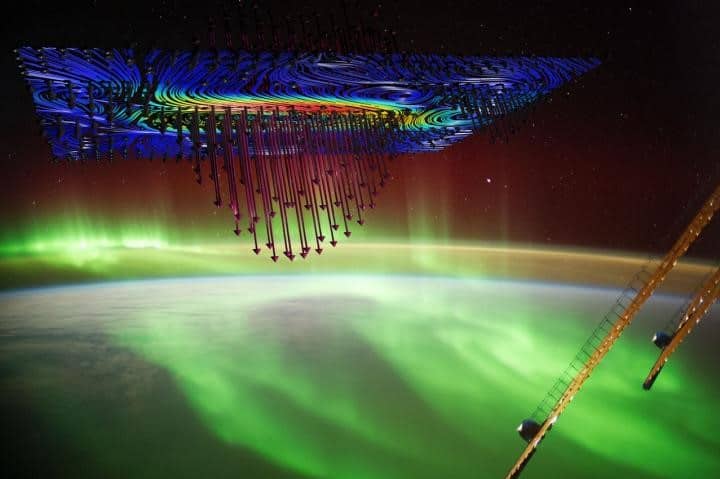
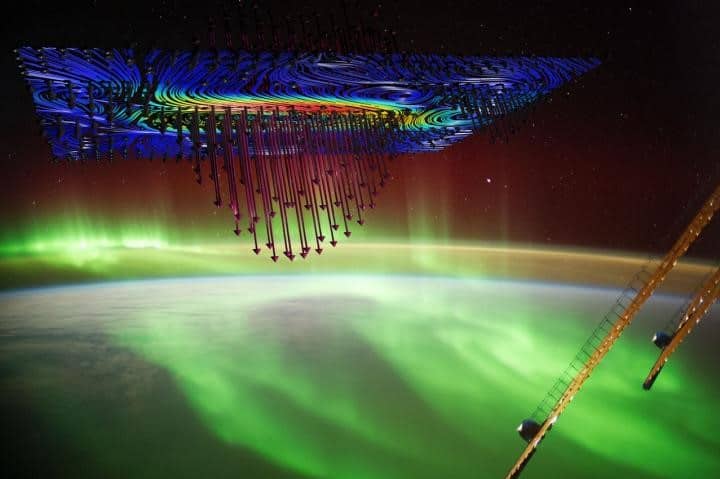
Plasma-β unity
Stefani and colleagues found that at up to 54 T, all measurements were dominated by the frequency of the alternating current. At precisely this point, however, a new signal with half that frequency appeared. This sudden period doubling, they explain, agrees perfectly with theoretical predictions of a mutual interaction with sound waves, indicating that the Alfvén waves had begun to travel at a faster-than-sound speed. This threshold, sometimes called plasma-β unity, is thought to play a key role in the corona heating.
Looking forward, the researchers, who report their work in Physical Review Letters, say they now plan to back up their experimental results with detailed numerical simulations. “These might, in turn, lead to improved designs and protocols of further experiments,” Stefani tells Physics World.

Isabelle Dumé is a contributing editor to Physics World.
from physicsworld.com 11/2/2022
Δεν υπάρχουν σχόλια:
Δημοσίευση σχολίου