Quantum sensor shrinks dark matter’s parameter space
07 Feb 2022
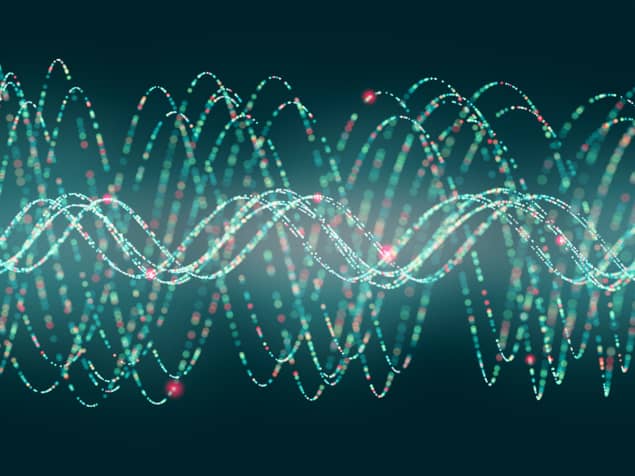
Physicists in Israel have extended the search for dark matter by using a new kind of quantum sensor to place stricter limits on so-called axion-like particles. They did so by monitoring the precession of xenon atoms and exploiting what are known as Floquet states to probe a greater mass range than was previously possible. They are now optimizing their experiment to further enhance sensitivities.
Dark matter is thought to make up around 85% of all the matter in the universe, but beyond its gravitational interactions scientists still have little idea about it composition. Having tried in vain to detect weakly interacting massive particles (WIMPs) for several decades, physicists are increasingly focusing their attention on axion-like particles (ALPs). These are a general type of dark matter that are inspired by the original axion, which was put forward in the 1970s as a means of solving a problem with the strong force. ALPs are spin-zero particles that could in principle possess any possible combination of mass and interaction strength.
Researchers have carried out numerous experiments looking for the signs of ALPs morphing into and out of photons – using what are known as haloscopes, helioscopes and “light shining through a wall” tests – to no avail. Others seek to detect ALPs’ effects on nuclei, including several groups that are trying to detect the oscillating magnetic fields that would be generated by the coupling between nuclei and extremely light ALPs.
Xenon and rubidium
In the latest work, Or Katz at the Weizmann Institute of Science in Rehovot, Israel and colleagues use a detector consisting of a magnetically shielded small glass cell filled with atoms of xenon-129 and rubidium-85. Two laser beams are directed at the cell – one a pump beam that polarizes the rubidium’s electron spin and with it the xenon’s nuclear spin, and the other a linearly-polarized probe beam that measures changes to the spins.
Going by the snappy name of Noble and Alkali Spin Detectors for Ultralight Coherent darK matter (NASDUCK), the experiment relies on the oscillating field of any passing axions rotating the collective xenon spin and thereby causing the nuclei to precess at the field’s oscillation frequency. That precession is measured thanks to the rubidium atoms imprinting their subsequent polarization on the probe beam, whose rotated polarization axis is picked up using a pair of photodiodes – in effect turning the rubidium into an optical magnetometer.
By exposing the atoms to a magnetic field along their initial spin axis, the precession of the xenon spins can be blocked for all but a very narrow oscillation frequency centred around the nuclear magnetic resonance (NMR) frequency of the applied field. Variations in the latter therefore allow the researchers to scan a range of oscillation frequencies – corresponding to a range of ALP masses – and measure the response of the rubidium atoms at each new setting.
Frequency mismatch
There is, however, a mismatch between the NMR frequency and the electron paramagnetic resonance (EPR) frequency – which determines the rubidium’s response. This discrepancy becomes more of a problem at higher ALP oscillation frequencies, and therefore at greater masses. It was to relieve this problem that the researchers employed a Floquet field – a strong magnetic field that also varies in time.
Floquet fields induce a temporary splitting of quantized energy levels and have been widely used to control material properties such as band structure and gyromagnetic ratios. In particular, Dmitry Budker of the University of Mainz in Germany and colleagues last year showed they could use this mechanism to convert a low-frequency magnetic field into a higher-frequency maser emission – and from the latter measure the former. Katz and colleagues have now applied a similar measurement scheme to their ALPs detector by using a Floquet field with a frequency about equal to the difference between the NMR and EPR frequencies to in effect close the gap between the two.
Nearly 3000 measurements
The researchers made nearly 3000 measurements over a period of five months, each time incrementing the NMR field by about 0.2 mG. This allowed them to make several scans of the dark-matter frequency band between 1–1000 Hz, corresponding to ALP masses between 4×10-15 eV and 4×10-12 eV. The search (unsurprisingly) yielded no signs of dark matter, but it did allow them to push down the upper limit on ALP-neutron coupling above 4×10-13 eV by about three orders of magnitude compared to previous terrestrial searches – thanks to the Floquet field.READ MORE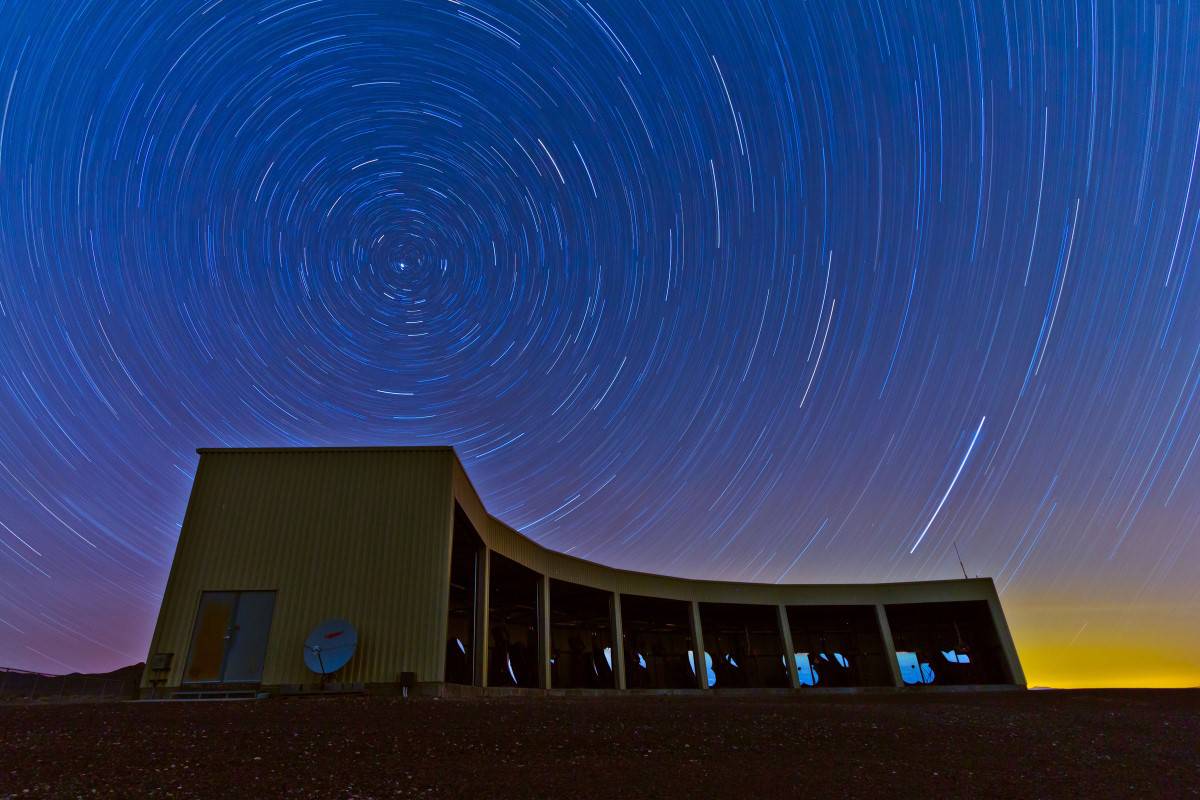
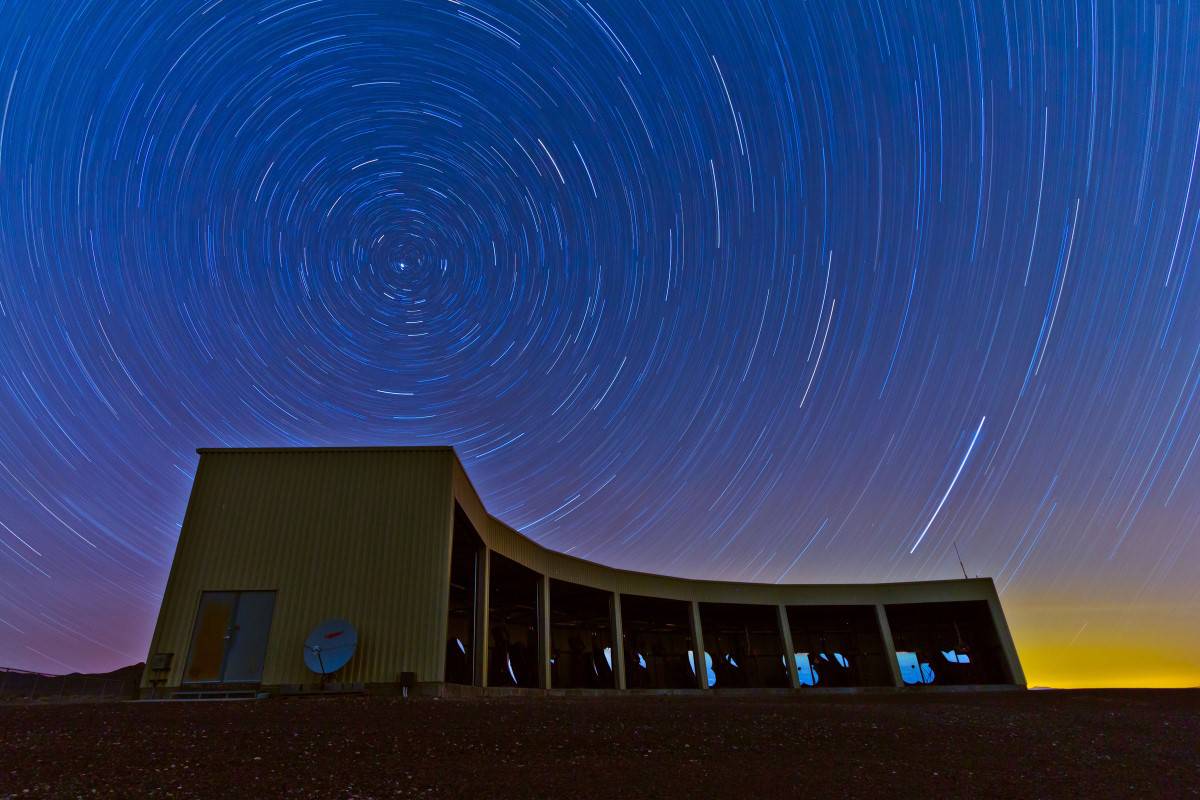
Katz and colleagues point out that still better limits have been set by astrophysical observations, but they argue that these are not as reliable as those derived from experiments on the ground. Observations involving the rate at which supernovae remnants cool, for example, rely on currently unknown mechanisms of supernova collapse. As such, they say, the resulting limits “should be taken with a grain of salt”.
In any case, the researchers are confident that they can beat the bounds from astrophysics – by pushing their own limits down a further two orders of magnitude or more. Such high sensitivities, they say, could in part be achieved by using low-noise ferrite shields to block stray magnetism and by sending the probe beam through the glass cell multiple times.
The research is described in Science Advances.
FROM PHYSICSWORLD.COM 11/2/2022
Δεν υπάρχουν σχόλια:
Δημοσίευση σχολίου