Structural batteries put multifunctional materials to the test
03 Feb 2022 Sponsored by TA Instruments
Designing structural components that can power an electric vehicle requires a comprehensive testing regime to assess the strength, stiffness and thermal stability of battery materials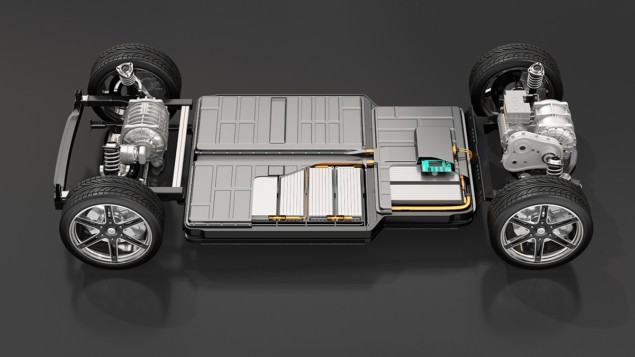
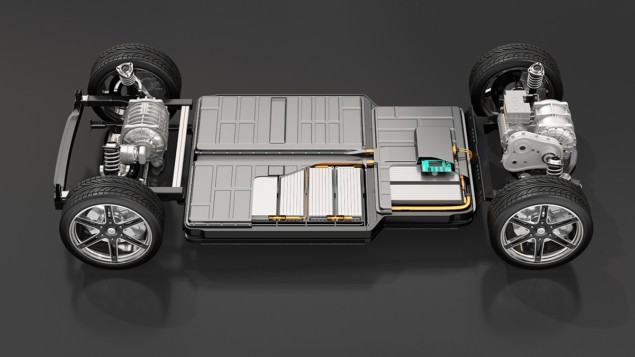
Ongoing advances in battery technology have for the first time made electric vehicles a viable alternative to cars powered by fossil fuels. However, further innovation will be crucial to drive widespread adoption of battery-powered vehicles – in particular to engineer improved energy-storage devices to allow drivers to travel further on a single charge – and for electrical solutions to be extended to other modes of transport, such as aviation.
One approach being explored by Jodie Lutkenhaus and her team at Texas A&M University is to design batteries and supercapacitors that could also become part of the vehicle’s load-bearing structure. Such structural energy-storage devices could provide more power without adding extra weight, improving the fuel efficiency of electric vehicles and potentially offering a secondary source of power for aircraft. “In a commercial airliner, for example, batteries embedded in the flooring could provide power for the touchscreens and other electrical equipment in the cabin,” explains Lutkenhaus. “Overall it could improve the fuel efficiency because you’re not using so much energy to power the electrical components.”
The multifunctional materials that are being developed for these structural devices must deliver a challenging combination of properties. Most electrode materials are powdery, with enough porosity to enable efficient ion transport, while structural components need to be stiff and strong. “We’re trying to create batteries and capacitors that mimic the properties of structural composites made from carbon-fibre reinforced epoxy,” explains Lutkenhaus. “We use additives like graphene and Kevlar nanofibres to improve the stiffness, but they are not very active. They dilute the battery-like behaviour in favour of the mechanical properties.”
Lutkenhaus and her team have tackled the problem through interfacial chemistry, enhancing the bonding between the additive and the electrode material to reduce the amount of additive needed to strengthen the material. In one recent research study, they demonstrated that supercapacitors made from reduced graphene oxide (rGO) could be strengthened by chemically modifying the structure using polydopamine, a highly adhesive polymer, and then adding just a small quantity of Kevlar nanofibres. “You can just sprinkle in just a little bit of the nanofibres and the mechanical properties go right up,” says Lutkenhaus.
Assessing the properties of these multifunctional materials requires a comprehensive suite of tests. To probe the mechanical properties of the electrodes, Lutkenhaus and team exploit a dynamic mechanical analyser (DMA) from TA Instruments, which is most commonly used to measure the changes in mechanical properties as the temperature is varied, or when stress or strain is applied. “We’re using it more as a tensile tester, which is a little unusual,” comments Lutkenhaus. “We use the DMA because the battery electrodes are very thin, around 100 microns or so. The DMA provides a thin-film and fibre-clamp arrangement that allows us to measure properties like modulus, toughness, and ultimate stress.”
Testing times: Jodie Lutkenhaus and her team exploit a dynamic mechanical analyser to measure the tensile strength of graphene electrodes reinforced with Kevlar nanofibers. (Courtesy: J Lutkenhaus)

The tests revealed that adding 10% by weight of Kevlar nanofibres to the modified rGO electrodes yields structures with a tensile strength of almost 200 MPa and a Young’s modulus in excess of 10 MPa – which is similar to the mechanical properties of natural materials such as wood or bone. Earlier work had required higher concentrations of nanofibres to achieve the same strength and stiffness, but in this study the chemical modification of the rGO electrodes increased the capacitance by 23% while still maintaining comparable mechanical properties.
Lutkenhaus and her team have also used Kevlar nanofibres to engineer battery separators, which provide a physical barrier between the anode and cathode to prevent short circuits but still need to allow ions to move between the two. “Battery separators are really difficult to design,” says Lutkenhaus. “They need a challenging combination of properties, including good ionic conductivity, mechanical robustness, and thermal stability over a wide temperature range.”
Lutkenhaus points out that the most common commercial separator used in lithium-ion batteries, called Celgard, starts to degrade at higher temperatures. “The material goes through a melting or softening transition that eventually causes the battery to fail,” she explains. “In our study we needed to identify any thermal transitions that would put a limit on the battery’s operating temperature.”
Lutkenhaus and her team compared the thermal properties of the standard Celgard separator with one based on Kevlar nanofibres. To measure thermal behaviour they used a differential scanning calorimeter (DSC) from TA Instruments, which at different temperatures measures the amount of heat needed to change the temperature of the material. As expected, the DSC profiles revealed that Celgard started to melt at around 160 °C, and on cooling recrystallized at 113 °C. In contrast, the Kevlar-based separator shows no change in thermal behaviour at temperatures of up to 400 °C. “It’s the unique case where you’re doing experiments in the hope of getting a flat line, because you don’t want to see any thermal signature,” says Lutkenhaus.
Other tests investigated the temperature at which the physical structure of the separators start to break down. Using a thermogravimetric analyser (TGA) from TA Instruments, which measures the mass of the sample as the temperature is increased, the researchers found that Celgard starts to lose mass at around 270 °C, while the Kevlar-based separator can be heated to almost 450 °C before showing any signs of degradation. Kevlar-based nanofibres were also found to self-extinguish when exposed to a flame – an important safety feature for batteries – while commercial Celgard versions shrank in dry conditions and burnt completely when wettened with an electrolyte.
Mechanical testing with a DMA show that in dry conditions the Kevlar-based separator has a Young’s modulus some 1000 times greater than that of the commercial Celgard version, and broadly equivalent strength and stiffness when wet. Meanwhile, creep measurements that probe the mechanical behaviour of the material under a constant stress reveal that the Celgard separator stretches out at higher temperatures, consistent with the melting and softening effect observed in its thermal profile. “The Kevlar-based separator does not show any deformation at temperatures of up to 400 °C, again showing better thermal stability than commercially available separators,” comments Lutkenhaus.
Lutkenhaus believes that the superior thermal properties of separators based on Kevlar nanofibres would make them suitable for energy storage devices operating in extreme environments. However, more work is needed to boost their electrochemical properties, since integrating the Kevlar-based separator into a lithium-ion battery resulted in a slightly reduced capacity compared to the Celgard version, as well a significant fall in capacity after 50 charge/discharge cycles.
Lutkenhaus and her team are also now investigating the potential of carbon fibre, commonly used in structural composites, to act as a mechanical matrix for battery materials. Embedding electrode materials into carbon fibre have been shown to yield devices that are stiff and strong, but do not yet store enough energy to rival standard lithium-ion batteries. “We also have a lot of interest in thermal behaviour because vehicles that need structural batteries might operate at lower temperatures or in a high-temperature desert,” says Lutkenhaus. “In addition, we need to start thinking about the effects of mechanical impact on these devices to understand how they might behave in an accident.”
TA Instruments is part of Waters Corporation, a specialty test and measurement company. More detailed information about the optimal testing solutions for battery materials and components can be found on the Waters/TA Instruments website.
Sponsored by TA Instruments
FROM PHYSICSWORLD.COM 4/2/2022
Δεν υπάρχουν σχόλια:
Δημοσίευση σχολίου